Exploring the Parallels Between Genome Editing and Assembly Coding
Written on
Chapter 1: Understanding Assembly Language
To initiate our exploration, let's define what assembly languages are. According to Investopedia, assembly languages are low-level programming languages that facilitate direct communication with a computer’s hardware. Unlike machine language, which comprises binary and hexadecimal codes, assembly languages are crafted for human readability.
Assembly languages serve as a bridge between machine code and human-readable languages. Programming in these languages necessitates a deeper level of critical thinking regarding how data is stored, accessed, and interpreted compared to higher-level languages.
The low-level nature of assembly languages offers specific advantages. For instance, they can be utilized to reverse engineer executables. This reverse engineering process involves deconstructing an executable to extract design details. Since the program has already been converted into machine code when reverse engineering occurs, the original high-level coding instructions are lost, leaving only machine code behind. Assembly languages, therefore, can modify executables and alter their functionalities.
Section 1.1: What Constitutes a Genome?
Turning our attention to genomic editing, this process operates on principles akin to those found in assembly language programming. Scientists employ various tools to alter the genomes of organisms. To grasp this fully, we need to briefly examine what genes are and their structure.
The National Human Genome Institute defines a gene as the fundamental unit of inheritance, passed from parents to offspring, containing the necessary information to determine traits. Genes are arranged sequentially on structures known as chromosomes, each of which contains a lengthy DNA molecule, only a portion of which corresponds to a single gene. Humans possess around 20,000 genes organized on their chromosomes.
Genes can be viewed as functions in computer science that contribute to building a program, with each function serving a unique role within the broader context of the program.
Another important aspect of genes is their composition. Genes are made up of DNA, which consists of sequences of nucleotides. There are only four primary nucleotides that form the building blocks of these sequences. Patterns such as the Pribnow box in prokaryotes and the TATAA box in eukaryotes emerge from these sequences.
Essentially, we can compare the nucleotides encoding genes to the binary numbers stored in a computer. Unlike binary, which has only two states (on and off), nucleotides can exhibit four states corresponding to the four types of nucleotides. Furthermore, recognizable patterns within sequences can be likened to assembly language instructions, where specific patterns represent the underlying nucleotide sequences.
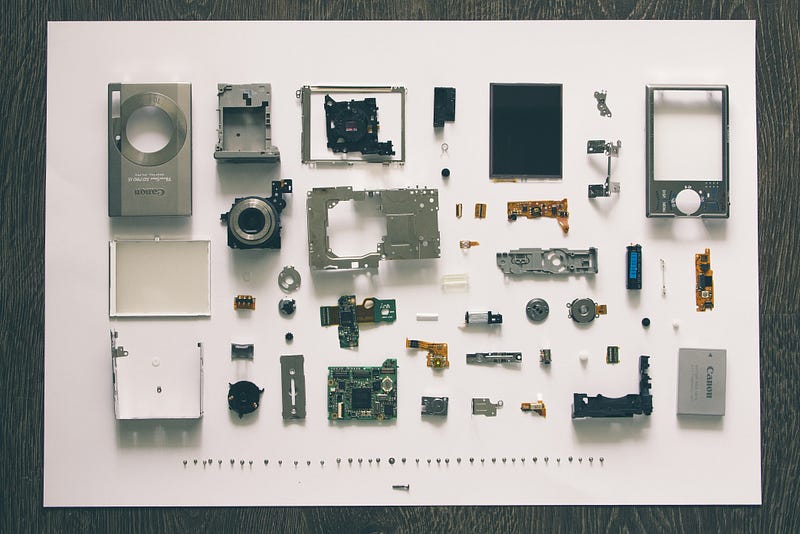
Section 1.2: Genome Editing as Reverse Engineering
Genome editing aims to fundamentally alter genes by directly targeting and modifying genomic sequences, mirroring the reverse engineering of programs through assembly languages. Both genome editing and reverse engineering focus on specific "machine code" sequences, modifying them to achieve desired results. In both scenarios, the specific sequence may be obscured—a phenomenon known in computer science as obfuscation—making it challenging to identify.
While a computer scientist might manually search through assembly code and possibly machine code to locate the necessary sequence, biologists can utilize biological methods to find their targets. In recent years, the CRISPR-Cas9 system has enabled scientists to pinpoint and safely edit specific sequences, opening up new possibilities.
Conclusion: The Future of Genome Editing
In conclusion, we have uncovered fascinating similarities between genome editing and assembly language programming. While significant progress is still needed to refine the science of genome editing, it represents a cutting-edge technology worth monitoring in the coming years.